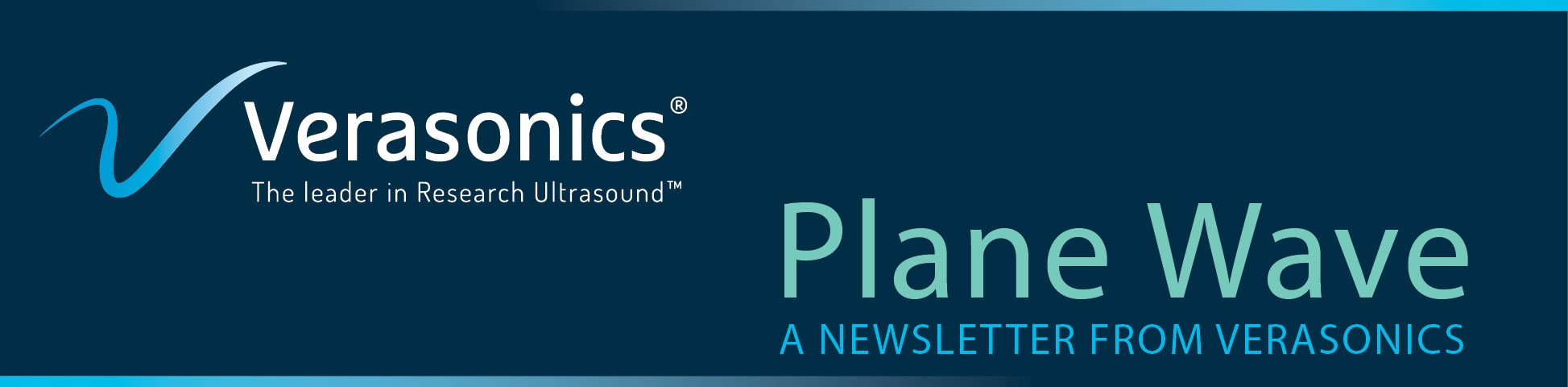
Clinical diagnostic ultrasound imaging typically operates in a range of 2-12 MHz. However, commercial manufacturers are now using higher frequencies (>12 MHz) with the advancement of smaller and more superficial applications, such as skin, eye and dental. In parallel, even higher frequency (30-100 MHz) ultrasound imaging capabilities have been developed specifically to image smaller structures of preclinical models. The use of high frequency ultrasound has grown with increased adoption and use of gene knockout and transgenic rodent models in research.
In addition to the innovative research capabilities of the trusted Vantage platform, the Vantage NXT Research Ultrasound System has the ability to access higher frequencies (30-60 MHz). This new architecture unlocks Vantage’s high-frame capabilities to transducers above 20 MHz center frequency, in addition to enhancing sensitivity.
High-frequency resolution and dynamic range are improved in both transmit and receive through 125 MHz 16-bit ADC, 2 ns timing precision and a minimum transmit pulse width of 4 ns. The patented NXT transceiver was engineered to provide industry-leading, transmit precision and linearity. This provides the performance necessary to enable techniques such as pulse compression, even at very high frequencies, improving SNR and penetration. Additionally, improvements in the hardware on Vantage NXT, modification to the software and the acquisitions sequences result in improved B-mode image quality.
The examples included here illustrate Vantage NXT’s high frequency capabilities to image rodent pre-clinical models.
Figure 1 illustrates increased spatial resolution and contrast of 30 MHz B-mode imaging of a contused rat spinal cord. Both cross-section and longitudinal images show the extent of a unilateral hematoma extending rostral/caudal and into midline respectively.
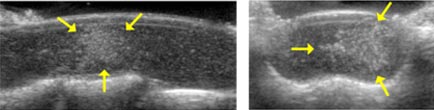
Figure 1 Contused rodent spinal cord injury model (a) longitudinal (b) cross-section. Courtesy: Zin Khaing/Matthew Bruce, University of Washington.
The upgraded receive path of Vantage NXT improves the sensitivity overall, most noticeably at higher frequencies. This development unlocks applications such as the study of blood flow. As is it well known, the backscatter from blood increases with frequency; and at 30 MHz, the blood flow signal approaches that of the background tissue. This enables the detection and separation of lower velocity flow, which is not possible at lower frequencies.
Figure 2a illustrates sub-centimeter per second flow in a mouse brain, which is not spatially resolved. This image is similar to what one would see with conventional contrast enhanced ultrasound (CEUS); however, in this acquisition, no injected ultrasound contrast agents (UCAs) were used to acquire the image. Figure 2b shows higher velocity flow in the larger spatially resolved vasculature, again without the use of UCAs. These images demonstrate the potential of Vantage NXT with its improved high frequency performance (increased sensitivity and dynamic range) to effectively image blood flow in preclinical models.
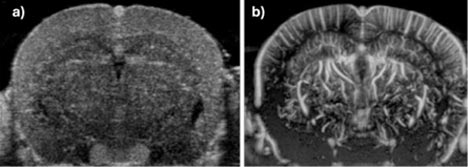
Figure 2 Blood flow in a mouse brain imaged at 30 MHz.
Courtesy: Pengfei Song/Matthew Bruce, University of Illinois/University of Washington.
Relatedly, ultrasound localized microscopy (ULM) has been used in the past decade to study the morphology of the vascular tree, particularly in the small (and hard to image) vessels. This technique uses microbubble UCAs to overcome the diffraction imaging limit and generate ultrasound images at the micron level. As the Vantage NXT platform now has a sampling frequency of 125 MHz compared to 62.5 MHz in the Vantage System, Vantage NXT can now be used with high frequency transducer (e.g. the Vermon L35-16vX transducer) without the need to use interleaved or bandwidth sampling. Therefore, Vantage NXT supports high frame rate acquisitions at 30 MHz, with also improved sensitivity over its predecessor, the Vantage platform.
Figure 3b illustrates the potential improvement of small vessel visualization with ULM with Vantage NXT relative to the previous Vantage system in Figure 3a. These images were acquired on a murine model using the L35-16vX transducer.
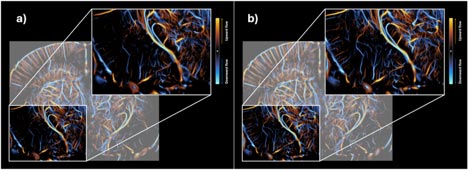
Figure 3: Comparative ULM imaging of mouse brain vasculature with the (a) Vantage (b) and Vantage NXT. Courtesy: Pengfei Song, University of Illinois.
Other potential applications of contrast imaging are to study the flow in cancerous masses and abdominal organs. The following images depict a mouse kidney, both its morphology and vascularization after an injection of contrast agent.
Figure 4a shows a 30 MHz cross-sectional B-mode image of the mouse kidney. Figure 4b illustrates the wash-in of a microbubble bolus highlighting the microvasculature. Figure 4c shows the power Doppler image of the higher velocity blood flow in the larger vasculature.
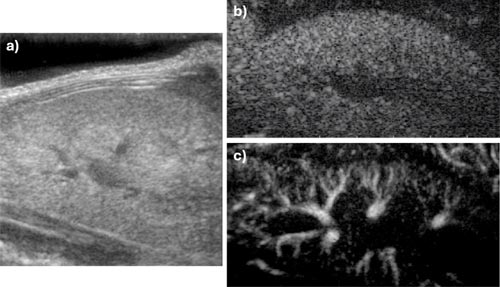
Figure 4: Mouse kidney imaged with L35-16vX (a) Cross sectional 30 MHz B-mode image with Vantage NXT (b) Long axis bolus wash-in of microbubbles. (c) Color flow image of larger vasculature. Courtesy: Mike Averkiou/Matthew Bruce University of Washington.
Lastly, functional ultrasound (fUS) is a area with growing momentum in research and preclinical use, with marked advantages in sensitivity, temporal resolution, cost and portability over MRI. Over the past decade this technique has been applied from rat models to clinical translation with human subjects. However, fUS imaging in a murine model has been challenging due to the high frequency and high frame rate requirements to sufficiently detect neural activity. The Vantage NXT platform unlocks these types of applications by sampling RF signals at 125 MHz and allowing ultra-fast acquisitions up to 20 to 30 kHz (limited only by the speed of sound in tissue).
Figure 5 illustrates changes in blood flow in the primary somatosensory area during whisker stimulation on a murine model.
